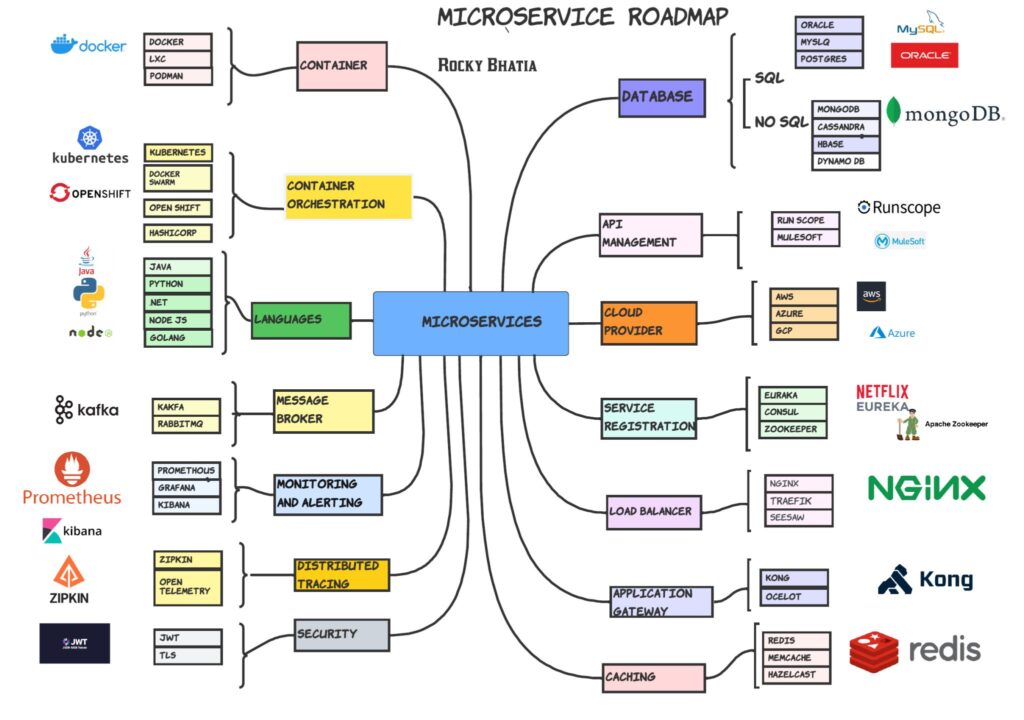
Learning
Critical Thinking
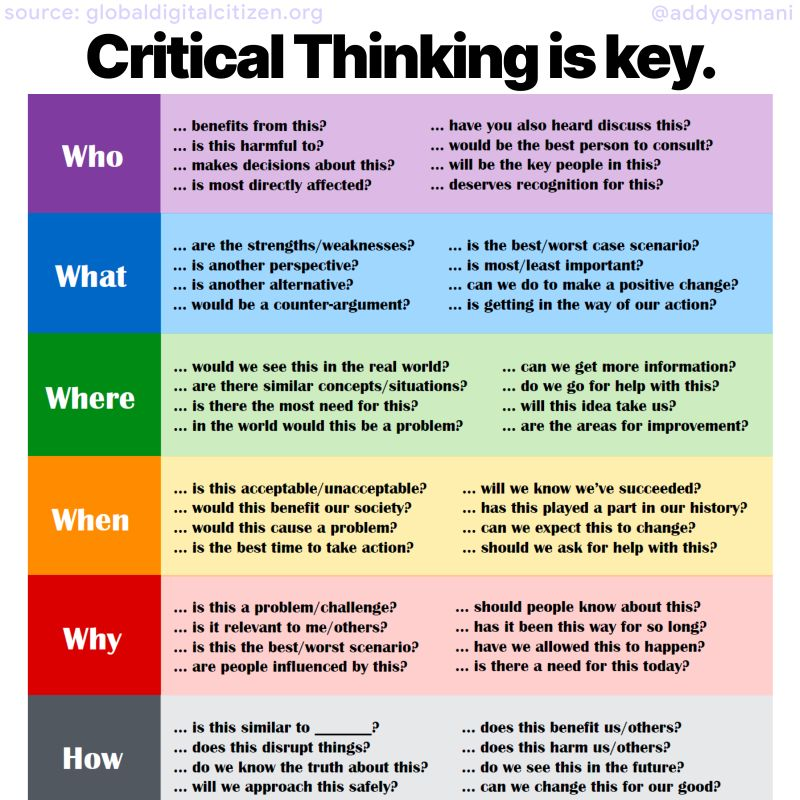
Tip: Critical thinking skills can be really valuable for Software engineers, Product and many other walks of life. It’s about approaching new information with a mix of humble curiosity and doubt.
Think independently and ask good questions that help make thoughtful decisions.
In broad strokes, some of the questions I like to ask based on critical thinking are:
➡️ How do we know we’re solving the right problem?
➡️ How do we know we’re solving the problem in the right way? (i.e. balancing rigor and efficiency, given our understanding of the problem and constraints)
➡️ If we don’t know the sources of our problem, how can we determine the root cause?
➡️ How can we break the key question down into smaller questions that we can analyze further?
➡️ Once we have one or more hypotheses, how do we structure work to evaluate them?
➡️ What shortcuts might we take if we’re under constraints (time pressure) without unduly compromising our analytics rigor around the question?
➡️ Does the evidence sufficiently support the conclusions?
How do we know when we are done? When is the solution “good enough”?
➡️ How do I communicate the solution clearly and logically to all stakeholders?
I’ve found these questions often help. Sometimes we’ll address the symptom of a problem, only to discover there are other symptoms that pop up. At other times, we might quickly ship a solution that creates more problems later down the road.
With a lens on critical thinking, we might challenge assumptions, look closer at the risk/benefit, seek out contradictory evidence, evaluate credibility and look for more data to build confidence we are doing the right thing.
Being in engineering or product, we can sometimes rush to solve a problem right away so it feels like we’re making progress or looks like we’re being responsive to stakeholders. This can introduce risks if we aren’t asking the right questions before doing so, fully considering causes and consequences. Put another way, critical thinking is thinking on purpose and forming your own conclusions. This goal-directed thinking can help you focus on root-cause issues that avoid future problems that arise from not keeping in mind causes and consequences.
Critical thinkers:
➡️ Raise mindful questions, formulating them clearly and precisely
➡️ Collect and assess relevant information, validating how they might answer the question
➡️ Arrive at well-reasoned conclusions and solutions, testing them against relevant criteria and standards
➡️ Think open mindedly within alternative systems of thought, recognizing and assessing, as need be, their assumptions, implications, and practical consequences
➡️ Communicate effectively with others in figuring out solutions to complex problems
Why i switched form docker desktop to Colima
DDEV is an open source tool that makes it simple to get local PHP development environments up and running within minutes. It’s powerful and flexible as a result of its per-project environment configurations, which can be extended, version controlled, and shared. In short, DDEV aims to allow development teams to use containers in their workflow without the complexities of bespoke configuration.
DDEV replaces more traditional AMP stack solutions (WAMP, MAMP, XAMPP, and so on) with a flexible, modern, container-based solution. Because it uses containers, DDEV allows each project to use any set of applications, versions of web servers, database servers, search index servers, and other types of software.
In March 2022, the DDEV team announced support for Colima, an open source Docker Desktop replacement for macOS and Linux. Colima is open source, and by all reports it’s got performance gains over its alternative, so using Colima seems like a no-brainer.
Migrating to Colima
First off, Colima is almost a drop-in replacement for Docker Desktop. I say almost because some reconfiguration is required when using it for an existing DDEV project. Specifically, databases must be reimported. The fix is to first export your database, then start Colima, then import it. Easy.
Colima requires that either the Docker or Podman command is installed. On Linux, it also requires Lima.
Docker is installed by default with Docker Desktop for macOS, but it’s also available as a stand-alone command. If you want to go 100% pure Colima, you can uninstall Docker Desktop for macOS, and install and configure the Docker client independently. Full installation instructions can be found on the DDEV docs site.
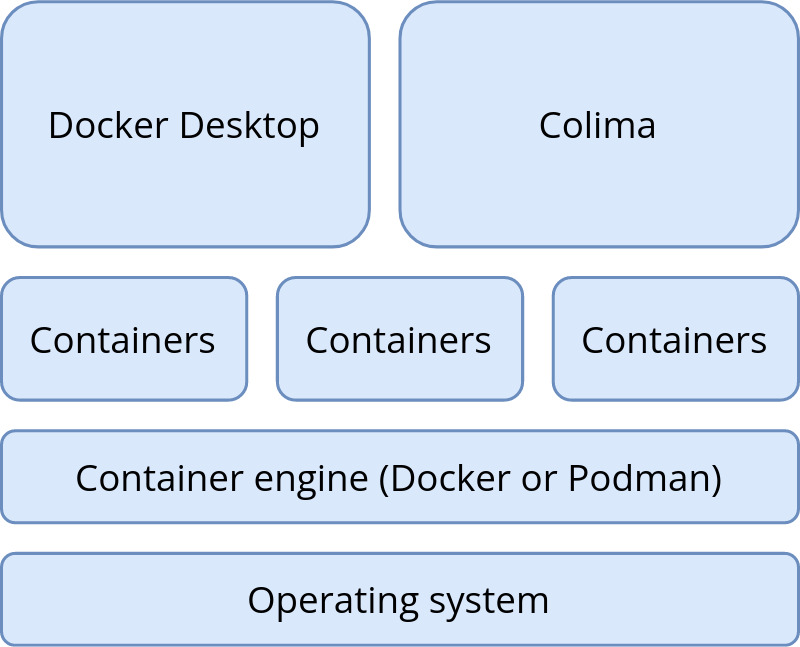
(Mike Anello,CC BY-SA 4.0)
If you choose to keep using both Colima and Docker Desktop, then when issuing docker commands from the command line, you must first specify which container you want to work with. More on this in the next section.
More on Kubernetes
Free online course: Containers, Kubernetes and Red Hat OpenShift technical over…
eBook: Storage Patterns for Kubernetes
An introduction to enterprise Kubernetes
How to explain Kubernetes in plain terms
eBook: Running Kubernetes on your Raspberry Pi homelab
eBook: A guide to Kubernetes for SREs and sysadmins
Install Colima on macOS
I currently have some local projects using Docker, and some using Colima. Once I understood the basics, it’s not too difficult to switch between them.
- To get started, install Colima using Homebrew
brew install colima
ddev poweroff
(just to be safe)- Next, start Colima with
colima start --cpu 4 --memory 4.
The--cpu
and--memory
options only have to be done once. After the first time, onlycolima start
is necessary. - If you’re a DDEV user like me, then you can spin up a fresh Drupal 9 site with the usual
ddev
commands (ddev config, ddev start
, and so on.) It’s recommended to enable DDEV’s mutagen functionality to maximize performance.
Switching between a Colima and Docker Desktop
If you’re not ready to switch to Colima wholesale yet, it’s possible to have both Colima and Docker Desktop installed.
- First, poweroff ddev:
ddev poweroff
- Then stop Colima:
colima stop
- Now run
docker context use default
to tell the Docker client which container you want to work with. The namedefault
refers to Docker Desktop for Mac. Whencolima start
is run, it automatically switches Docker to thecolima
context. - To continue with the default (Docker Desktop) context, use the
ddev start
command.
Technically, starting and stopping Colima isn’t necessary, but the ddev poweroff
command when switching between two contexts is.
Recent versions of Colima revert the Docker context back to default
when Colima is stopped, so the docker context use default
command is no longer necessary. Regardless, I still use docker context show
to verify that either the default
(Docker Desktop for Mac) or colima
context is in use. Basically, the term context
refers to which container provider the Docker client routes commands to.
Try Colima
Overall, I’m liking what I see so far. I haven’t run into any issues, and Colima-based sites seem a bit snappier (especially when DDEV’s Mutagen functionality is enabled). I definitely foresee myself migrating project sites to Colima over the next few weeks.
Luigi è a lavoro ?
Se nei giorni in cui lavoro da remoto entra qualcuno in ufficio chiedendo di me, ecco non dite mai “No, oggi Luigi non c’è, è in smartworking” ma rispondete “Sì, sì, oggi Luigi c’è, lavora in smartworking”.
Si potrebbe sintetizzare così lo smartworking, in italiano il lavoro agile. Perché si lavora anche così, non necessariamente seduti alla scrivania nel proprio ufficio. L’abbiamo imparato, chi già non lo faceva prima, in questi due anni di pandemia. Ci si può organizzare meglio, si risparmia tempo e tanto la produttività quanto la vita personale ne traggono vantaggio. Win-win, per stare sull’inglese. Che poi, per rispondere agli scettici, guardate che chi non lavora da casa, di regola non lavora neppure dall’ufficio.
Dal 1 settembre, ormai ci siamo, torna obbligatorio l’accordo individuale, sospeso negli ultimi due anni. Cos’è? È il fulcro dello smartworking (che ricordiamo non è un contratto di lavoro ma una sua modalità di esecuzione).
Datore di lavoro e lavoratore si devono mettere d’accordo su come organizzare il lavoro, in parte all’interno dei locali aziendali e in parte all’esterno, senza precisi vincoli di orario e di luogo di lavoro. È un accordo, il che significa che se una delle parti non è – appunto – d’accordo non se ne fa nulla. Ed è individuale, non collettivo (anche se spesso regolamenti o contratti collettivi possono dare indicazioni).
L’accordo deve essere stipulato in forma scritta, dicono le norme, “ai fini della regolarità amministrativa e della prova” e deve essere conservato dal datore di lavoro per cinque anni dalla sua sottoscrizione. Nessuna sanzione esplicita se manca l’accordo, ma conseguenze in base ai fini che è chiamato a raggiugere. Come provare i contenuti dell’accordo? Quali le conseguenze in caso di infortunio?
Novità degli ultimi giorni: l’accordo individuale, a differenza del periodo pre-pandemico, non deve più essere trasmesso al Ministero del Lavoro al quale basterà sapere (oltre all’elenco dei lavoratori interessati) la data di sottoscrizione dell’accordo e la sua durata. La comunicazione di questi dati (che poi il Ministero trasmetterà all’Inail) dovrà essere effettuata entro cinque giorni dalla sottoscrizione dell’accordo, pena una sanzione pecuniaria da 100 a 500 euro. Per la prima fase transitoria il termine è fissato al 1 novembre.
Bene, inizia la fase due dello smartworking ordinario e non più emergenziale!
E ricordatevi che risposta dare quando, non trovandomi in ufficio, vi chiederanno “Oggi Luigi è al lavoro?”.
backup restore mysql docker
# Backup docker exec CONTAINER /usr/bin/mysqldump -u root --password=root DATABASE > backup.sql # Restore cat backup.sql | docker exec -i CONTAINER /usr/bin/mysql -u root --password=root DATABASE
Every Linux networking tool i know
Migrate databases to Kubernetes using Konveyor
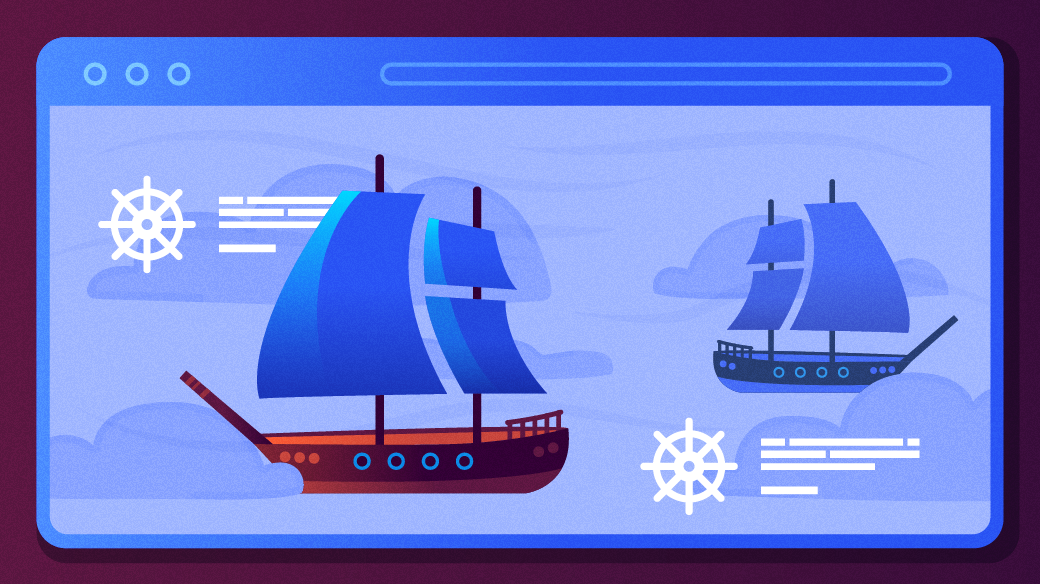
Kubernetes Database Operator is useful for building scalable database servers as a database (DB) cluster. But because you have to create new artifacts expressed as YAML files, migrating existing databases to Kubernetes requires a lot of manual effort. This article introduces a new open source tool named Konveyor Tackle-DiVA-DOA (Data-intensive Validity Analyzer-Database Operator Adaptation). It automatically generates deployment-ready artifacts for database operator migration. And it does that through datacentric code analysis.
What is Tackle-DiVA-DOA?
Tackle-DiVA-DOA (DOA, for short) is an open source datacentric database configuration analytics tool in Konveyor Tackle. It imports target database configuration files (such as SQL and XML) and generates a set of Kubernetes artifacts for database migration to operators such as Zalando Postgres Operator.
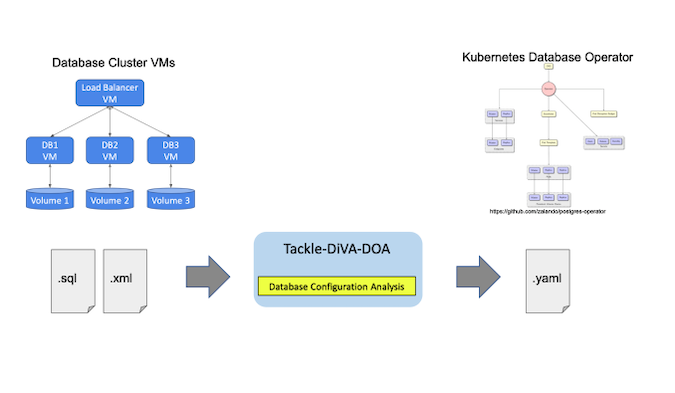
DOA finds and analyzes the settings of an existing system that uses a database management system (DBMS). Then it generates manifests (YAML files) of Kubernetes and the Postgres operator for deploying an equivalent DB cluster.
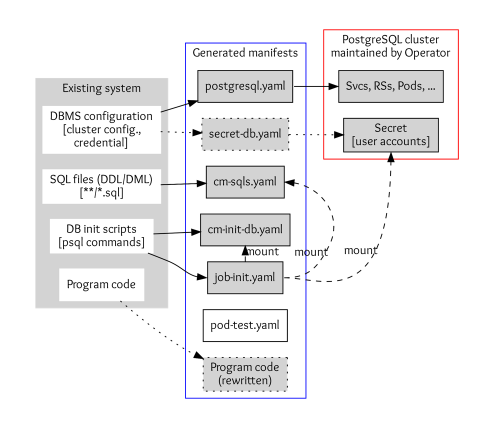
Database settings of an application consist of DBMS configurations, SQL files, DB initialization scripts, and program codes to access the DB.
- DBMS configurations include parameters of DBMS, cluster configuration, and credentials. DOA stores the configuration to
postgres.yaml
and secrets tosecret-db.yaml
if you need custom credentials.
- SQL files are used to define and initialize tables, views, and other entities in the database. These are stored in the Kubernetes ConfigMap definition
cm-sqls.yaml
.
- Database initialization scripts typically create databases and schema and grant users access to the DB entities so that SQL files work correctly. DOA tries to find initialization requirements from scripts and documents or guesses if it can’t. The result will also be stored in a ConfigMap named
cm-init-db.yaml
.
- Code to access the database, such as host and database name, is in some cases embedded in program code. These are rewritten to work with the migrated DB cluster.
Tutorial
DOA is expected to run within a container and comes with a script to build its image. Make sure Docker and Bash are installed on your environment, and then run the build script as follows:
$ cd /tmp
$ git clone https://github.com/konveyor/tackle-diva.git
$ cd tackle-diva/doa
$ bash util/build.sh
…
docker image ls diva-doa
REPOSITORY TAG IMAGE ID CREATED SIZE
diva-doa 2.2.0 5f9dd8f9f0eb 14 hours ago 1.27GB
diva-doa latest 5f9dd8f9f0eb 14 hours ago 1.27GB
This builds DOA and packs as container images. Now DOA is ready to use.
The next step executes a bundled run-doa.sh
wrapper script, which runs the DOA container. Specify the Git repository of the target database application. This example uses a Postgres database in the TradeApp application. You can use the -o
option for the location of output files and an -i
option for the name of the database initialization script:
$ cd /tmp/tackle-diva/doa
$ bash run-doa.sh -o /tmp/out -i start_up.sh \
https://github.com/saud-aslam/trading-app
[OK] successfully completed.
The /tmp/out/
directory and /tmp/out/trading-app
, a directory with the target application name, are created. In this example, the application name is trading-app
, which is the GitHub repository name. Generated artifacts (the YAML files) are also generated under the application-name directory:
$ ls -FR /tmp/out/trading-app/
/tmp/out/trading-app/:
cm-init-db.yaml cm-sqls.yaml create.sh* delete.sh* job-init.yaml postgres.yaml test//tmp/out/trading-app/test:
pod-test.yaml
The prefix of each YAML file denotes the kind of resource that the file defines. For instance, each cm-*.yaml
file defines a ConfigMap, and job-init.yaml
defines a Job resource. At this point, secret-db.yaml
is not created, and DOA uses credentials that the Postgres operator automatically generates.
Now you have the resource definitions required to deploy a PostgreSQL cluster on a Kubernetes instance. You can deploy them using the utility script create.sh
. Alternatively, you can use the kubectl create
command:
$ cd /tmp/out/trading-app
$ bash create.sh # or simply “kubectl apply -f .”configmap/trading-app-cm-init-db created
configmap/trading-app-cm-sqls created
job.batch/trading-app-init created
postgresql.acid.zalan.do/diva-trading-app-db created
The Kubernetes resources are created, including postgresql
(a resource of the database cluster created by the Postgres operator), service
, rs
, pod
, job
, cm
, secret
, pv
, and pvc
. For example, you can see four database pods named trading-app-*
, because the number of database instances is defined as four in postgres.yaml
.
$ kubectl get all,postgresql,cm,secret,pv,pvc
NAME READY STATUS RESTARTS AGE
…
pod/trading-app-db-0 1/1 Running 0 7m11s
pod/trading-app-db-1 1/1 Running 0 5m
pod/trading-app-db-2 1/1 Running 0 4m14s
pod/trading-app-db-3 1/1 Running 0 4mNAME TEAM VERSION PODS VOLUME CPU-REQUEST MEMORY-REQUEST AGE STATUS
postgresql.acid.zalan.do/trading-app-db trading-app 13 4 1Gi 15m RunningNAME TYPE CLUSTER-IP EXTERNAL-IP PORT(S) AGE
service/trading-app-db ClusterIP 10.97.59.252 <none> 5432/TCP 15m
service/trading-app-db-repl ClusterIP 10.108.49.133 <none> 5432/TCP 15mNAME COMPLETIONS DURATION AGE
job.batch/trading-app-init 1/1 2m39s 15m
Note that the Postgres operator comes with a user interface (UI). You can find the created cluster on the UI. You need to export the endpoint URL to open the UI on a browser. If you use minikube, do as follows:
$ minikube service postgres-operator-ui
Then a browser window automatically opens that shows the UI.
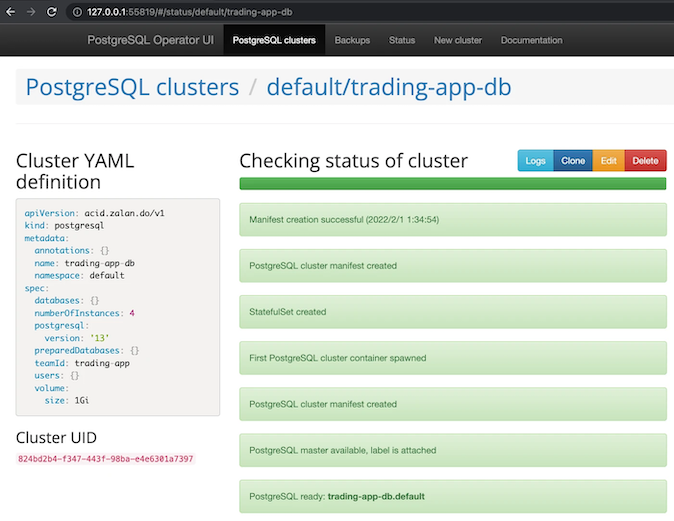
(Yasuharu Katsuno and Shin Saito, CC BY-SA 4.0)
Now you can get access to the database instances using a test pod. DOA also generated a pod definition for testing.
$ kubectl apply -f /tmp/out/trading-app/test/pod-test.yaml # creates a test Pod
pod/trading-app-test created
$ kubectl exec trading-app-test -it -- bash # login to the pod
The database hostname and the credential to access the DB are injected into the pod, so you can access the database using them. Execute the psql
metacommand to show all tables and views (in a database):
# printenv DB_HOST; printenv PGPASSWORD
(values of the variable are shown)# psql -h ${DB_HOST} -U postgres -d jrvstrading -c '\dt'
List of relations
Schema | Name | Type | Owner
--------+----------------+-------+----------
public | account | table | postgres
public | quote | table | postgres
public | security_order | table | postgres
public | trader | table | postgres
(4 rows)# psql -h ${DB_HOST} -U postgres -d jrvstrading -c '\dv'
List of relations
Schema | Name | Type | Owner
--------+-----------------------+------+----------
public | pg_stat_kcache | view | postgres
public | pg_stat_kcache_detail | view | postgres
public | pg_stat_statements | view | postgres
public | position | view | postgres
(4 rows)
After the test is done, log out from the pod and remove the test pod:
# exit
$ kubectl delete -f /tmp/out/trading-app/test/pod-test.yaml
Finally, delete the created cluster using a script:
$ bash delete.sh
Why http 3.0 ?
Selecting Performance Monitoring Tools
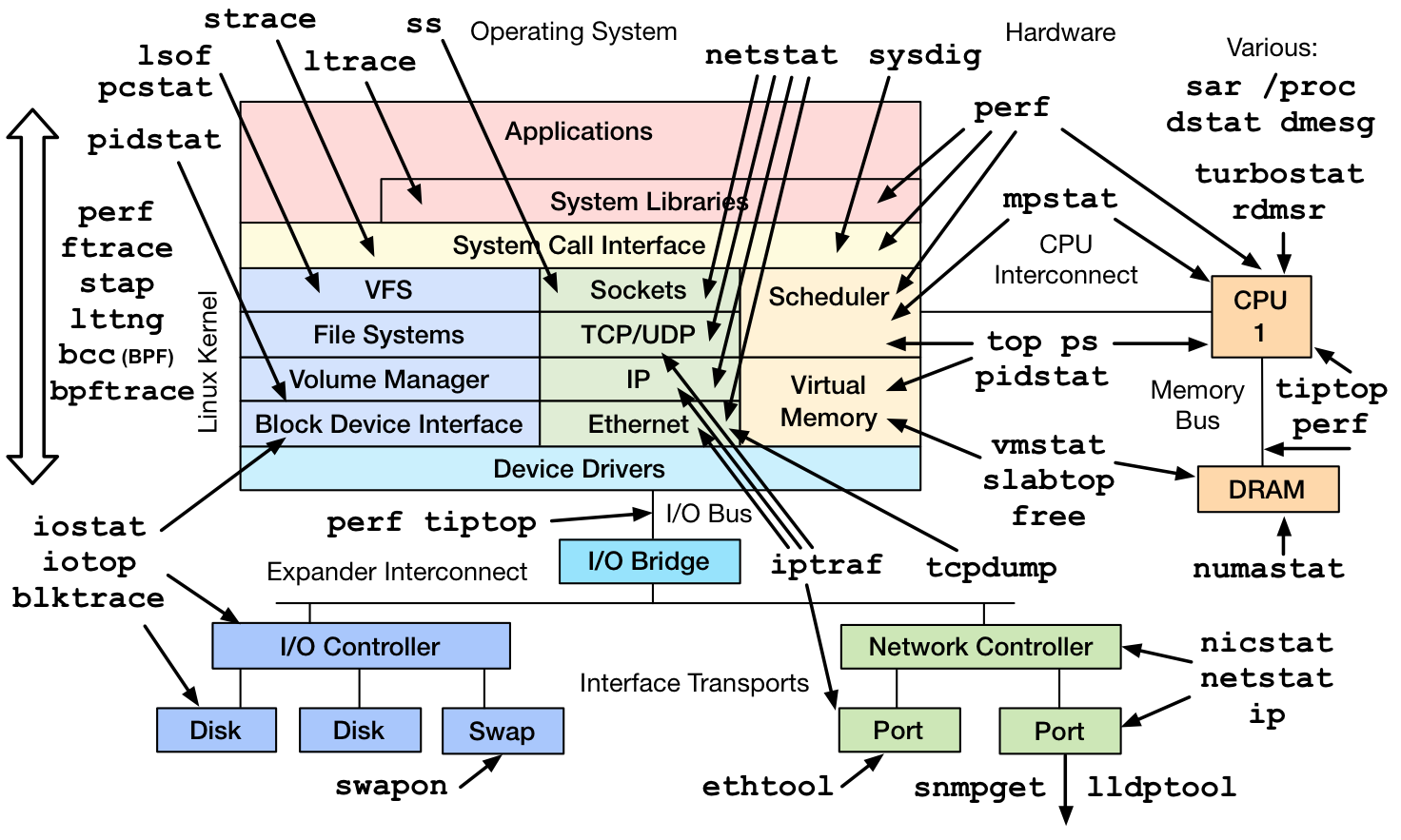
System monitoring is a helpful approach to provide the user with data regarding the actual timing behavior of the system. Users can perform further analysis using the data that these monitors provide. One of the goals of system monitoring is to determine whether the current execution meets the specified technical requirements.
These monitoring tools retrieve commonly viewed information, and can be used by way of the command line or a graphical user interface, as determined by the system administrator. These tools display information about the Linux system, such as free disk space, the temperature of the CPU, and other essential components, as well as networking information, such as the system IP address and current rates of upload and download.
Monitoring Tools
The Linux kernel maintains counterstructures for counting events, that increment when an event occurs. For example, disk reads and writes, and process system calls, are events that increment counters with values stored as unsigned integers. Monitoring tools read these counter values. These tools provide either per process statistics maintained in process structures, or system-wide statistics in the kernel. Monitoring tools are typically viewable by non-privileged users. The ps and top commands provide process statistics, including CPU and memory.
Monitoring Processes Using the ps Command
Troubleshooting a system requires understanding how the kernel communicates with processes, and how processes communicate with each other. At process creation, the system assigns a state to the process.
Use the ps aux command to list all users with extended user-oriented details; the resulting list includes the terminal from which processes are started, as well as processes without a terminal. A ?
sign in the TTY
column represents that the process did not start from a terminal.
[user@host]$
ps aux
USER PID %CPU %MEM VSZ RSSTTY
STAT START TIME COMMAND user 1350 0.0 0.2 233916 4808 pts/0 Ss 10:00 0:00 -bash root 1387 0.0 0.1 244904 2808?
Ss 10:01 0:00 /usr/sbin/anacron -s root 1410 0.0 0.0 0 0 ? I 10:08 0:00 [kworker/0:2... root 1435 0.0 0.0 0 0 ? I 10:31 0:00 [kworker/1:1... user 1436 0.0 0.2 266920 3816 pts/0 R+ 10:48 0:00 ps aux
The Linux version of ps supports three option formats:
- UNIX (POSIX) options, which may be grouped and must be preceded by a dash.
- BSD options, which may be grouped and must not include a dash.
- GNU long options, which are preceded by two dashes.
The output below uses the UNIX options to list every process with full details:
[user@host]$
ps -ef
UID PID PPID C STIME TTY TIME CMD root 2 0 0 09:57 ? 00:00:00 [kthreadd] root 3 2 0 09:57 ? 00:00:00 [rcu_gp] root 4 2 0 09:57 ? 00:00:00 [rcu_par_gp] ...output omitted...
Key Columns in ps OutputPID
This column shows the unique process ID.TIME
This column shows the total CPU time consumed by the process in hours:minutes:seconds format, since the start of the process.%CPU
This column shows the CPU usage during the previous second as the sum across all CPUs expressed as a percentage.RSS
This column shows the non-swapped physical memory that a process consumes in kilobytes in the resident set size, RSS
column.%MEM
This column shows the ratio of the process’ resident set size to the physical memory on the machine, expressed as a percentage.
Use the -p
option together with the pidof command to list the sshd
processes that are running.
[user@host ~]$
ps -p $(pidof sshd)
PID TTY STAT TIME COMMAND 756 ? Ss 0:00 /usr/sbin/sshd -D [email protected]... 1335 ? Ss 0:00 sshd: user [priv] 1349 ? S 0:00 sshd: user@pts/0
Use the following command to list of all processes sorted by memory usage in descending order:
[user@host ~]$
ps ax --format pid,%mem,cmd --sort -%mem
PID %MEM CMD 713 1.8 /usr/libexec/sssd/sssd_nss --uid 0 --gid 0 --logger=files 715 1.8 /usr/libexec/platform-python -s /usr/sbin/firewalld --nofork --nopid 753 1.5 /usr/libexec/platform-python -Es /usr/sbin/tuned -l -P 687 1.2 /usr/lib/polkit-1/polkitd --no-debug 731 0.9 /usr/sbin/NetworkManager --no-daemon ...output omitted...
Various other options are available for ps including the o
option to customize the output and columns shown.
Monitoring Process Using top
The top command provides a real-time report of process activities with an interface for the user to filter and manipulate the monitored data. The command output shows a system-wide summary at the top and process listing at the bottom, sorted by the top CPU consuming task by default. The -n 1
option terminates the program after a single display of the process list. The following is an example output of the command:
[user@host ~]$
top -n 1
Tasks: 115 total, 1 running, 114 sleeping, 0 stopped, 0 zombie %Cpu(s): 0.0 us, 3.2 sy, 0.0 ni, 96.8 id, 0.0 wa, 0.0 hi, 0.0 si, 0.0 st MiB Mem : 1829.0 total, 1426.5 free, 173.6 used, 228.9 buff/cache MiB Swap: 0.0 total, 0.0 free, 0.0 used. 1495.8 avail Mem PID USER PR NI VIRT RES SHR S %CPU %MEM TIME+ COMMAND 1 root 20 0 243968 13276 8908 S 0.0 0.7 0:01.86 systemd 2 root 20 0 0 0 0 S 0.0 0.0 0:00.00 kthreadd 3 root 0 -20 0 0 0 I 0.0 0.0 0:00.00 rcu_gp ...output omitted...
Useful Key Combinations to Sort FieldsRES
Use Shift+M to sort the processes based on resident memory.PID
Use Shift+N to sort the processes based on process ID.TIME+
Use Shift+T to sort the processes based on CPU time.
Press F and select a field from the list to use any other field for sorting.
IMPORTANT
The top command imposes a significant overhead on the system due to various system calls. While running the top command, the process running the top command is often the top CPU-consuming process.
Monitoring Memory Usage
The free command lists both free and used physical memory and swap memory. The -b
, -k
, -m
, -g
options show the output in bytes, KB, MB, or GB, respectively. The -s
option is passed as an argument that specifies the number of seconds between refreshes. For example, free -s 1 produces an update every 1 second.
[user@host ~]$
free -m
total used free shared buff/cache available Mem: 1829 172 1427 16 228 1496 Swap: 0 0 0
The near zero values in the buff/cache
and available
columns indicate a low memory situation. If the available memory is more than 20% of the total, and the used memory is close to the total memory, then these values indicate a healthy system.
Monitoring File System Usage
One stable identifier that is associated with a file system is its UUID, a very long hexadecimal number that acts as a universally unique identifier. This UUID is part of the file system and remains the same as long as the file system is not recreated. The lsblk -fp command lists the full path of the device, along with the UUIDs and mount points, as well as the type of file system in the partition. If the file system is not mounted, the mount point displays as blank.
[user@host ~]$
lsblk -fp
NAME FSTYPE LABEL UUID MOUNTPOINT /dev/vda ├─/dev/vda1 xfs 23ea8803-a396-494a-8e95-1538a53b821c /boot ├─/dev/vda2 swap cdf61ded-534c-4bd6-b458-cab18b1a72ea [SWAP] └─/dev/vda3 xfs 44330f15-2f9d-4745-ae2e-20844f22762d / /dev/vdb └─/dev/vdb1 xfs 46f543fd-78c9-4526-a857-244811be2d88
The findmnt command allows the user to take a quick look at what is mounted where, and with which options. Executing the findmnt command without any options lists out all the mounted file systems in a tree layout. Use the -s
option to read the file systems from the /etc/fstab
file. Use the -S
option to search the file systems by the source disk.
[user@host ~]$
findmnt -S /dev/vda1
TARGET SOURCE FSTYPE OPTIONS / /dev/vda1 xfs rw,relatime,seclabel,attr2,inode64,noquota
The df command provides information about the total usage of the file systems. The -h
option transforms the output into a human-readable form.
[user@host ~]$
df -h
Filesystem Size Used Avail Use% Mounted on devtmpfs 892M 0 892M 0% /dev tmpfs 915M 0 915M 0% /dev/shm tmpfs 915M 17M 899M 2% /run tmpfs 915M 0 915M 0% /sys/fs/cgroup /dev/vda1 10G 1.5G 8.6G 15% / tmpfs 183M 0 183M 0% /run/user/1000
The du command displays the total size of all the files in a given directory and its subdirectories. The -s
option suppresses the output of detailed information and displays only the total. Similar to the df -h command, the -h
option displays the output into a human-readable form.
[user@host ~]$
du -sh /home/user
16K /home/user
Using GNOME System Monitor
The System Monitor available on the GNOME desktop provides statistical data about the system status, load, and processes, as well as the ability to manipulate those processes. Similar to other monitoring tools, such as the top, ps, and free commands, the System Monitor provides both the system-wide and per-process data. These monitoring tools retrieve commonly viewed information, and can be used by way of the command line or a graphical user interface, as determined by the system administrator. Use the gnome-system-monitor command to access the application from a command terminal.
To view the CPU usage, go to the Resources tab and look at the CPU History chart.
![]() |
Figure 2.2: CPU usage history in System Monitor
The virtual memory is the sum of the physical memory and the swap space in a system. A running process maps the location in physical memory to files on disk. The memory map displays the total virtual memory consumed by a running process, which determines the memory cost of running that process instance. The memory map also displays the shared libraries used by the process.
![]() |
Figure 2.3: Memory map of a process in System Monitor
To display the memory map of a process in System Monitor, locate a process in the Processes tab, right-click a process in the list, and select Memory Maps.